ALL >> General >> View Article
Mitochondrial Dysfunction In Cardiovascular Disease
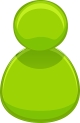
Introduction
Mitochondria are essential organelles responsible for the production of cellular energy in the form of adenosine triphosphate (ATP) through oxidative phosphorylation (OXPHOS). The heart, due to its continuous contractile activity, has a high energy demand and is critically dependent on mitochondrial function for normal physiological and pathological processes. Mitochondrial dysfunction has emerged as a central mechanism in the pathogenesis of cardiovascular diseases (CVDs), including ischemic heart disease, heart failure, hypertension, and arrhythmias. This technical overview discusses the molecular mechanisms of mitochondrial dysfunction in cardiovascular disease, its impact on cellular and organ function, and the potential therapeutic strategies to mitigate mitochondrial-related pathophysiology in CVDs.
Mitochondrial Function in Cardiovascular Cells
Mitochondria are highly dynamic organelles that perform several key functions crucial for the health of cardiovascular cells. They are involved in:
ATP Production via Oxidative Phosphorylation: In the mitochondria, energy production is driven ...
... by the electron transport chain (ETC), which is composed of complex I-IV embedded in the inner mitochondrial membrane. Electrons derived from NADH and FADH2 produced during the citric acid cycle are transferred through these complexes to ultimately reduce oxygen to water at complex IV. This electron transfer drives proton pumps that create an electrochemical gradient (proton motive force) across the inner mitochondrial membrane, which is utilized by ATP synthase (complex V) to produce ATP.
Calcium Homeostasis: Mitochondria play a crucial role in buffering intracellular calcium concentrations. They take up calcium from the cytoplasm in response to cellular signaling and help maintain cellular homeostasis by storing calcium in the matrix and releasing it when required for cellular signaling. Dysregulation of mitochondrial calcium handling can lead to pathophysiological conditions such as mitochondrial permeability transition (MPT) and cell death.
Reactive Oxygen Species (ROS) Production: Mitochondria are the primary source of ROS due to the incomplete reduction of oxygen molecules during electron transport in the ETC. Under normal conditions, low levels of ROS act as signaling molecules. However, excessive ROS generation due to mitochondrial dysfunction can cause oxidative stress, which damages cellular components such as lipids, proteins, and mitochondrial DNA (mtDNA), contributing to the pathogenesis of cardiovascular diseases.
Apoptosis and Cell Death: Mitochondria are central regulators of apoptosis. The release of pro-apoptotic factors such as cytochrome c from the mitochondrial intermembrane space into the cytoplasm triggers caspase activation, leading to programmed cell death. Mitochondrial dysfunction in cardiovascular tissues can lead to inappropriate cell death, contributing to the progression of CVDs.
Molecular Mechanisms of Mitochondrial Dysfunction in Cardiovascular Disease
Mitochondrial dysfunction in cardiovascular disease can result from several factors, including oxidative damage, altered mitochondrial dynamics, mutations in mitochondrial DNA, and defects in mitochondrial signaling. Below are the primary molecular mechanisms contributing to mitochondrial dysfunction in cardiovascular pathologies:
1. Oxidative Stress and ROS Accumulation
Excessive ROS generation is a hallmark of mitochondrial dysfunction and a major contributor to cardiovascular disease progression. Under normal conditions, the ETC produces ROS as a byproduct of electron transfer; however, under pathological conditions such as ischemia, hypoxia, or heart failure, there is an increase in mitochondrial ROS production. This increase is due to the altered electron flow through the ETC, particularly at complex I and III, which results in the incomplete reduction of oxygen.
The accumulation of ROS causes oxidative damage to mitochondrial lipids, proteins, and mtDNA. For instance, lipid peroxidation of mitochondrial membranes leads to membrane destabilization and disruption of mitochondrial function. ROS also modify proteins involved in mitochondrial dynamics and bioenergetics, impairing the capacity of mitochondria to generate ATP. Furthermore, oxidative damage to mtDNA leads to mutations that compromise the mitochondrial respiratory chain complexes, creating a vicious cycle of mitochondrial dysfunction.
2. Mitochondrial Permeability Transition (MPT) and Calcium Overload
Mitochondrial permeability transition is a critical event in mitochondrial dysfunction. The opening of the mitochondrial permeability transition pore (mPTP) occurs when the inner mitochondrial membrane becomes permeable to ions and small molecules, disrupting the electrochemical gradient required for ATP production. Under pathological conditions such as ischemia-reperfusion injury, excessive ROS and calcium overload activate the mPTP, leading to mitochondrial swelling, loss of membrane potential, and the release of pro-apoptotic factors (e.g., cytochrome c), triggering cell death.
Calcium overload plays a significant role in mitochondrial dysfunction. During stress conditions like ischemia, excessive intracellular calcium is taken up by mitochondria, causing mitochondrial matrix expansion and rupture of the mitochondrial membrane. This exacerbates cellular injury and promotes cell death pathways in the myocardium, contributing to myocardial infarction and heart failure.
3. Mitochondrial Dynamics Dysregulation
Mitochondrial dynamics refer to the continuous processes of mitochondrial fusion and fission that maintain mitochondrial quality and function. In response to cellular stress, mitochondria can undergo fission to segregate damaged components or fusion to promote functional compensation. Mitochondrial fission is regulated by dynamin-related protein 1 (DRP1), while fusion is mediated by mitofusins (MFN1 and MFN2) and optic atrophy 1 (OPA1). In cardiovascular diseases, this dynamic balance is often disrupted, leading to mitochondrial fragmentation, reduced mitochondrial function, and increased susceptibility to apoptosis.
In heart failure, for example, the upregulation of DRP1 and downregulation of fusion proteins contribute to mitochondrial fragmentation, reduced ATP production, and elevated ROS levels. This dysfunction is exacerbated by altered signaling pathways, including those associated with autophagy (mitophagy), which is responsible for removing damaged mitochondria. Dysfunctional mitophagy further impairs mitochondrial quality control, worsening cardiac injury.
4. Mitochondrial DNA Mutations
Mitochondrial DNA is more prone to mutations than nuclear DNA due to its proximity to the ETC and lack of protective histones. In cardiovascular diseases, mutations in mtDNA contribute to defective mitochondrial function. For example, mutations in genes encoding subunits of the OXPHOS complexes (such as ATP6, ND1, or CYTB) lead to impaired ATP synthesis and defective mitochondrial bioenergetics, contributing to myocardial ischemia and heart failure.
Mitochondrial mutations may also affect the regulation of ROS production and the activation of apoptotic pathways, accelerating tissue damage and organ dysfunction.
Therapeutic Approaches Targeting Mitochondrial Dysfunction in Cardiovascular Disease
Given the critical role of mitochondria in cardiovascular disease, several therapeutic strategies have been developed to target mitochondrial dysfunction and restore normal mitochondrial function. These include:
1. Mitochondrial Antioxidants
Mitochondrial-targeted antioxidants, such as MitoQ, MitoTEMPO, and SkQ1, have been developed to specifically target ROS within mitochondria. These compounds aim to reduce oxidative stress, limit mitochondrial damage, and improve mitochondrial function. Clinical studies are ongoing to assess the efficacy of these antioxidants in reducing myocardial injury and improving outcomes in heart failure and ischemic heart disease.
2. Mitochondrial Biogenesis Activation
Stimulating mitochondrial biogenesis to increase the number of functional mitochondria is another potential therapeutic strategy. Activators of peroxisome proliferator-activated receptor gamma coactivator 1-alpha (PGC-1α), a key regulator of mitochondrial biogenesis, are being investigated as potential treatments for heart failure. Exercise training is a natural way to activate PGC-1α and increase mitochondrial function, which has been shown to improve cardiac outcomes in patients with heart failure.
3. MPTP Inhibition
Inhibitors of the mPTP, such as cyclosporine A, have been studied for their potential to prevent ischemia-reperfusion injury by inhibiting pore opening. By preserving mitochondrial integrity, these inhibitors may help reduce myocardial damage and improve survival after myocardial infarction.
4. Gene Therapy and Mitochondrial Transplantation
Gene therapy approaches, including the use of CRISPR/Cas9 to correct mitochondrial DNA mutations, hold promise in treating mitochondrial diseases. Additionally, mitochondrial transplantation, where healthy mitochondria are delivered to damaged cardiac cells, is an emerging area of research, with the potential to restore mitochondrial function and improve heart function in patients with severe myocardial injury.
Conclusion
Mitochondrial dysfunction plays a central role in the pathogenesis of cardiovascular diseases, contributing to impaired ATP production, increased ROS production, and cell death. Understanding the molecular mechanisms underlying mitochondrial dysfunction provides critical insights into the development of novel therapeutic strategies. Approaches targeting mitochondrial biogenesis, oxidative stress, mitochondrial dynamics, and mPTP inhibition offer promising avenues for the treatment of cardiovascular diseases and could lead to more effective management of conditions such as heart failure, ischemic heart disease, and hypertension. However, further research and clinical trials are needed to fully elucidate the potential of these therapeutic strategies in improving cardiovascular health.
kEYWORDS
Add Comment
General Articles
1. Metal Roofing San Antonio: Durable, Efficient, And StylishAuthor: Hasan Hes
2. Free Roof Inspection San Antonio: Protect Your Home Today!
Author: Hasan Hes
3. Book Nonstop Flights On Delta Airlines Online
Author: Delta Phone Number
4. Purchase 2 & 3 Bhk Flats In Rishita Mulberry Heights—luxurious Living Space In Lucknow
Author: Star Estate
5. Bramha Isle Of Life: Redefining Urban Luxury And Investment
Author: Armaan
6. Menlo # Studio: Raising Urban Living In Pune's It Hub
Author: Armaan
7. The Power Of Subscription Models In Online Selling: How To Set Up And Scale
Author: Yash Kumar
8. The Good Life (eudaimonia): Introductory Overview
Author: Chaitanya Kumari
9. Best Astrologer In Tennessee
Author: Master Ji
10. Nicotine Pouches Manufacturers In India: The Rise Of Organic And Natural Ingredients
Author: Zvol
11. Buy 4 Bhk Flats In Purvanchal Royal Atlantis—lucknow Luxury Residence
Author: Star Estate
12. Best Spiritual Healer In Cayman Islands
Author: Cayman Islands
13. How To Play Slope Game: A Complete Guide For Beginners
Author: Emily Johnson
14. Instagram Growth Hacks: Stand Out In A Crowded Space
Author: valana
15. What Is The Difference Between Belief And Knowledge?
Author: Chaitanya Kumari